Hyperspectral Imaging: Making the Invisible Visible
The human eye can perceive only a small portion of the entire electromagnetic spectrum: the visible light range that extends from roughly the 380 nanometer (nm) wavelength to 700 nm. The rest of the spectrum is invisible to humans, although we encounter other wavelengths every day—from the ultraviolet (UV) light of the sun that causes our skin to tan or burn, to the microwave ovens we use to heat our food.
Advancements in imaging system technology, starting with the discovery of X-rays in 1895, now enable us to “see” virtually the entire range of electromagnetic light energy, from high-energy, short-wavelength gamma rays to low-energy, long-wavelength radio waves. One newer imaging technique is hyperspectral imaging, which was developed only within the last 30 years.
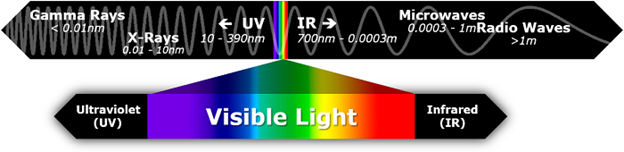
The full spectrum of electromagnetic radiation. The visible light range—what humans see—is only a narrow slice in the middle of the spectrum.
What Is Hyperspectral Imaging?
In broad terms, hyperspectral imaging refers to the capture and processing of images incorporating a very large number of wavelengths including both visible and invisible areas of the spectrum. Different materials—from rocks and chemical compunds to vegetation and human tissues—each have slightly different spectral characteristics, called a spectral “fingerprint.” Thus, analysis of spectral images can show us information about the world around us that we could not discern with the naked eye.
More precisely called spectral imaging or spectral analysis, the technology was refined by NASA in the 1980s as part of its Airborne Imaging Spectrometer (AIS) initiative. An early test of the technology was for geological analysis of rock formations using data collected by flying over an area of Nevada.1
Compared to visual imaging systems (such as cameras), which might capture and evaluate an image in three wavelength bands (red, blue, green), or multispectral imaging systems, which might measure the RGB bands plus near-infrared (NIR), hyperspectral imaging systems capture an image that encompasses a wider spectrum of wavelengths broken down into tens or even hundreds of bands.
For example, the US Geological Survey (USGS) collected hyperspectral data in Afghanistan in 2007 to generate detailed maps of surface mineral distribution. They were able to identify and map 31 different classes of materials by combining data “collected over a series of 28 flights, using four different modules in one instrument to cover a spectral range of 0.43 to 2.48 microns (430 nm to 2480 nm). Images were processed over 128 color bands, each 14-20 nm wide.”2
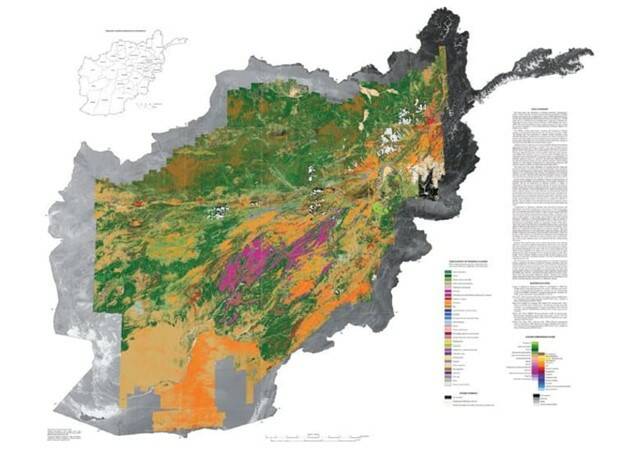
Mineral deposit map of Afghanistan complied from hyperspectral image data by the USGS. (Image Source: USGS)
As with any digital camera, hyperspectral systems rely on a sensor to capture information, with higher resolution sensors generally preferred to capture more data per pixel. Typically, light waves entering the system are directed through a narrow slit, and then separated into different wavelengths using a diffraction grating.
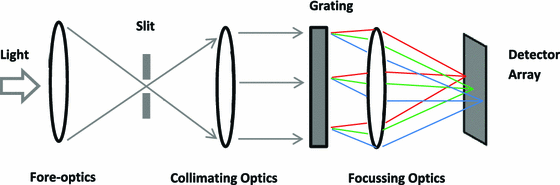
Schematic of how a typical hyperspectral imaging camera works. Light enters at left, is focused through an entrance slit, then directed through a diffraction grating to separate the wavelengths, which are then focused toward and captured by a sensor (detector array). (Image: Source)
Different techniques can be used to scan an area to capture images: spatial scanning, spectral scanning, non-scanning, and spatiospectral scanning. Each scanning approach uses a slightly different version of the above schematic and yields different output.
For example, spatial scanning typically incorporates a prism, and spectral scanning might use optical band-pass filters. Many of today’s hyperspectral imaging systems offer at least 100 spectral bands, with a variety of wavelength ranges available for different applications, for example 1-5 nm, 400-1000 nm, etc.3
Applications of Hyperspectral Imaging
The number of current and potential applications of hyperspectral imaging is vast. With drone technology, it is now easier than ever to send imaging systems into remote or difficult-to-reach areas to collect information.
In fact, hyperspectral remote sensing systems have already been used for “spaceborne, airborne, and ground-based sensing, unmanned aerial systems, and underwater vehicle-based“4 exploration. Some of the many application areas include agriculture, astronomy, biomedical imaging, environmental monitoring, food processing, geology, mineralogy, molecular biology, physics, and surveillance. Just a few examples from different industries:
- Agriculture. Hyperspectral data collected by aircraft can be used to analyze crops to characterize growth, molecular composition, water levels, invasive weeds, and much more.
- Food Processing. Food products such as fruit can be assessed to identify any quality issues such as bruises or rotten spots; systems can characterize the grade, marbling, color, and texture of meats; or systems can be used to sort nuts from shell fragments and any foreign particles.
- Environment. Algae blooms or contamination in lakes and rivers can be easily detected. Hyperspectral imaging can identify the precise amounts of chemicals such as phosphates and nitrates in a body of water or wetlands.
- Mining & Fossil Fuels. Imaging systems can pinpoint mineral deposits and grade them with a high degree of precision, drastically improving the accuracy of exploratory drilling. They can also be used to identify and evaluate oil spills.
- Medical Diagnosis. Modern medical care and disease treatment relies heavily on advanced imaging technologies such as MRI, CT, and PET scanning to assess internal structures such as bone, tissues, and organs. Hyperspectral imaging is a valuable addition to this toolkit. It can often be more cost-effective, safe, and easy-to-use in a clinic setting5 to obtain high-resolution images of a patient’s tissue morphology (shape and structure) and biochemical features.
- Thin Film Inspection. Thin films are vital in many industries today, from the plastic wrap used to store food to the high-grade films used for TFT semiconductors. By projecting light through the film and measuring spectral interference, imaging systems can measure films down to the micron to ensure uniform thickness.
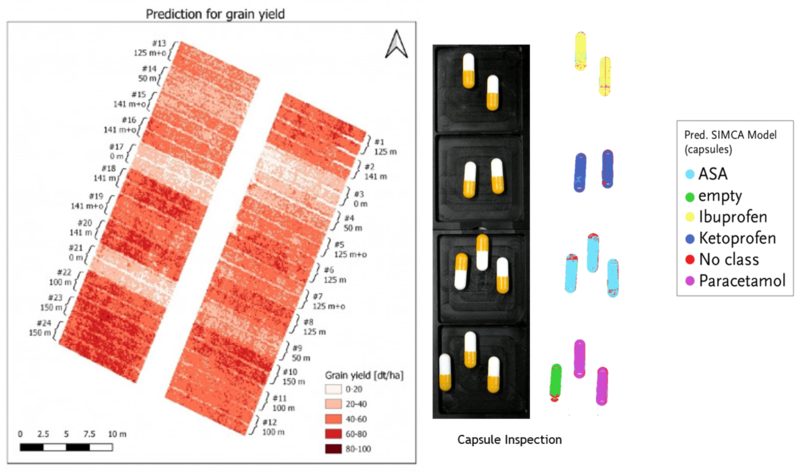
Left: Predicting yield in a wheat field as a function of fertilizer concentration. Chlorophyll was measured for 24 plots using 8 nitrogen treatments (Image Source). Right: Pharmaceuticals can be analyzed to verify ingredients and concentrations, check capsule and blister pack seals, and assess other quality specifications, even on high-speed production lines. (Image: © Specim)
Application Example: Specim Inline Industrial Inspection
One of Radiant’s sister companies in the Konica Minolta family is expert at hyperspectral imaging for both research and industrial use: Finland-based Specim Spectral Imaging, Ltd. Their systems are being used in multiple industrial settings, including recycling and waste management operations, which have become increasingly important on our crowded planet.
Efficiently recycling waste into reusable raw materials is an important step to help slow global warming and reduce exploitation of natural resources. To do this effectively, a recycling plant must be capable of separating a broad array of materials, reliably, and accurately. Material purity is also important to ensure trace amounts of different substances don’t contaminate materials being melted and processed for reuse.
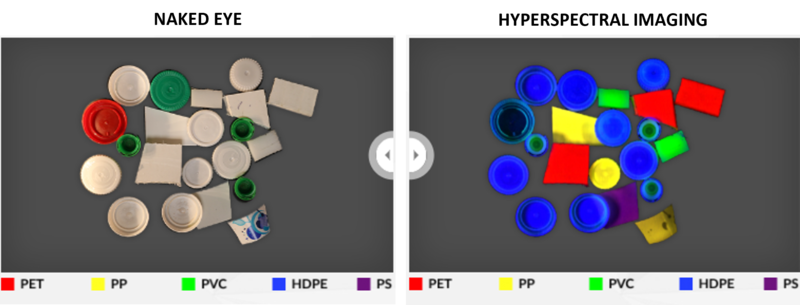
The naked eye can see the color of dye used to make a plastic part, but not much more (left). With a hyperspectral imaging system, each type of plastic and its chemical composition can be identified and measured (right). (Images: © Specim)
Specim’s hyperspectral sensor systems are helping recyclers to improve material handling and sorting, better enabling them to turn a profit. For example, the company helped Spanish firm PICVISA to use a machine vision approach using Specim’s compact FX17 Camera to sort 6000 kg of plastic packaging per hour. Read the Case Study or watch the video:
A Spectral Perspective
Although much of our planet’s Northern Hemisphere is experiencing some uncomfortably hot weather currently, we can still be grateful for the Sun, our largest source of electromagnetic energy. Beyond imaging systems, X-ray machines, cell phones, radar navigation, night vision goggles, UV disinfection, radio communication, and a host of other beneficial applications of the different spectra we can now use thanks to man-made energy sources, the Sun’s light has always been what makes life possible on this planet.
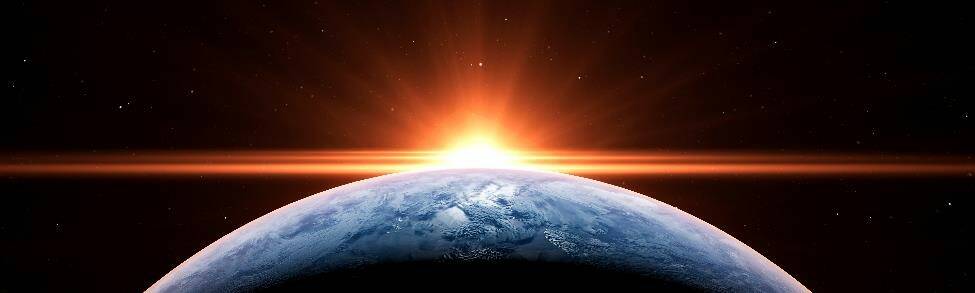
CITATIONS
- Feldman, S. C., Taranik, J. V. and Mouat, D. A., “A First Look at Airborne Imaging Spectrometer (AIS) Data in an Area of Altered Volcanic Rocks and Carbonate Formations, Hot Creek Range, South Central Nevada,” PL Proc. of the Airborne Imaging Spectrometer Data Anal. Workshop, June 15, 1985.
- Gibbons, K., “Hyperspectral Imaging What is it? How does it work?” Tech Briefs, March 1, 2014.
- Ibid.
- Lodhi, V., Chakravarty, D., and Mitra, P., “Hyperspectral Imaging for Earth Observation: Platforms and Instruments,” Journal of the Institute of Science, Vol 98, pp 429-443, May 9, 2018. DOI: 10.1007/s41745-018-0070-8
- Yoon, J., “Hyperspectral Imaging for Clinical Applications,” BioChip Journal, Vol 16, pp 1-12, January 4, 2022. DOI: 10.1007/s13206-021-00041-0
Join Mailing List
Stay up to date on our latest products, blog content, and events.
Join our Mailing List