Reaching the Full Color Potential of MicroLED Displays
As microLED display devices continue to move from prototypes toward broad commercialization, one of the challenges for developers is how to realize full-color displays. For a microLED display, each tiny individual LED (light emitting diode) acts as its own emitter, producing light independently. This enables microLEDs to deliver brightness and contrast that is especially desirable for applications such as automobile displays and AR/MR (augmented and mixed reality) headsets where displayed images compete with bright ambient lighting, such as sunlight.
Fabricating Color MicroLED Displays
MicroLED displays are constructed from tiny (<100 micrometer) LED chips, that reside on a display backplane (substrate + electronics). Monochrome LED chips are grown epitaxially, typically on a wafer. Thus, a full-color microLED display can be achieved via three primary methods:
- Integrating red, green, and blue (RGB) microLED chips onto the backplane by transferring them from the wafer and bonding;
- Adding a color conversion layer (such as quantum dots) on top of a mono-color or bi-color LED array; or
- Directly growing multi-color microLEDs on the same substrate.1
Numbers (1) and (2) have become common; number (3) has proven more challenging thus far except for in very small displays.2 Using method (1), RGB microLEDs can be assembled into matrices through the mass-transfer process to achieve full-color displays. “However, the mass-transfer process remains challenging owing to its low transfer yield, slow throughput, and high fabrication cost.”3 Some developers have opted for method (2), using color conversion based on a quantum dot layer over blue or UV microLEDs.
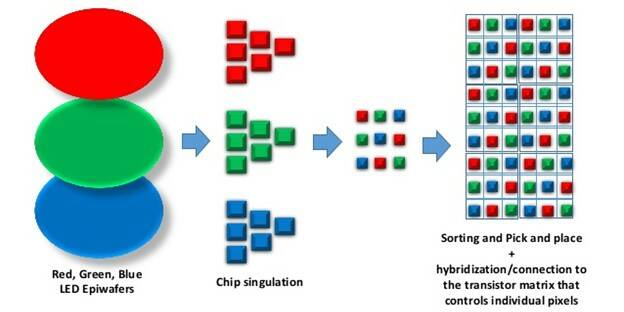
Representation of full-color microLED fabrication method (1): epitaxial growth of monochrome LED wafers, separated into chiplets, then transferred to a matrix to create an RGB display. (Image: Yole Développement).
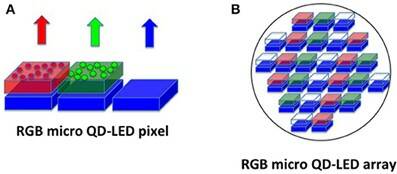
Representation of full-color microLED fabrication method (2): an RGB pixel is driven by three blue micro-LEDs covered with red and green quantum dots (left). A matrix of many blue micro-LEDs patterned with red and green quantum dots then forms a display (right).4 (Image: Source)
Any of these techniques has its potential advantages and drawbacks and one or more may emerge as a preferred method for commercial production. But at the end of each process, the result is the same: microLED displays that exhibit some degree of color non-uniformity, because of their independent emissions.
As display makers move towards mass production of microLED display devices, ensuring brightness and color uniformity can be extremely challenging. It is necessary to be able to measure and quantify every pixel and subpixel element of an emissive display to identify defects and ensure uniformity, to produce the level of quality that consumers expect.

Input signals for target color gamut of DCP-P3 (D65) (top row), compared to actual microLED display output (bottom row), where a dominant vertical pattern and block-wise stamp marks are clearly seen.5 (Images © Samsung Research / Samsung Electronics)
Quantifying Color as Perceived by Humans
In 1931, the Commission Internationale de L’éclairage (CIE) defined a standard for scientifically quantifying the physical properties of color as perceived by a human observer, enabling accurate mathematical representation and reproduction of those colors. To ensure accuracy when measuring highly variable microLEDs, a measurement system is needed that can discern color variation with equivalent sensitivity to the human eye. A standard human spectral sensitivity is quantified through mathematical formula of the CIE standard to provide chromaticity values as coordinates within the CIE color space.6
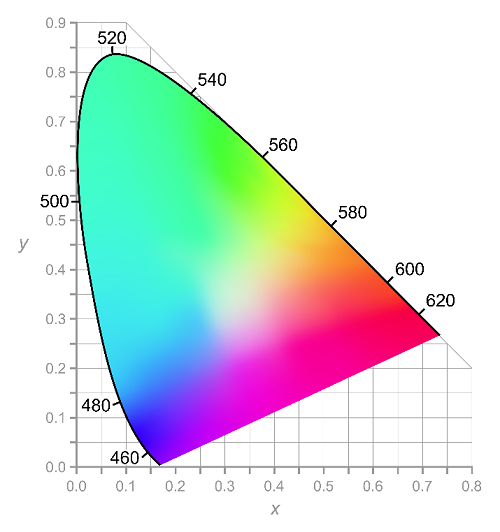
A graphical representation of color space in the CIE 1931 chromaticity diagram, including all colors visible to the human eye. The black numbers on the edge give the visible spectrum in wavelengths, while perceived colors are represented as coordinate points (x and y) within these limits.
For microLED display devices to achieve mass-market success, they must deliver a flawless consumer experience, including uniform color appearance. Defects, variations in color or brightness, and other irregularities can quickly deflate buyer satisfaction, hurt brand reputation, and erode market share. If these issues cannot be addressed and corrected at the component level, low yields and high production costs will impede the viability of microLED display technologies for mass production and market commercialization.
Measuring MicroLED Display Chromaticity
High-resolution tristimulus imaging colorimeter systems such as ProMetric® I-Series Imaging Colorimeters provide an efficient quality control solution to support production benchmarks for microLED displays. These systems rely on their color filter method, calibrations, and subpixel measurement capabilities to ensure accurate data is captured at the pixel and subpixel level, thus enabling display correction that increases yields and safeguards manufacturing resources.
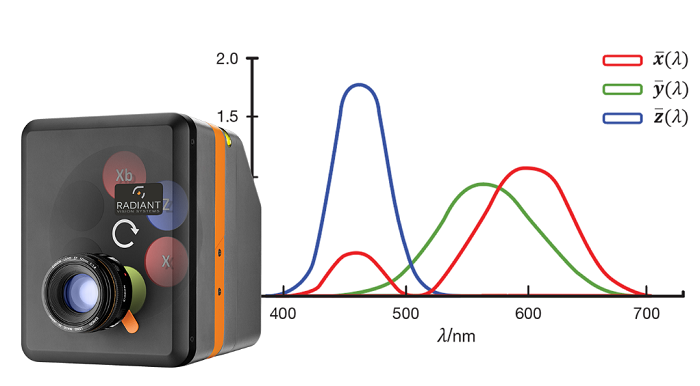
A CIE-matched tristimulus filter wheel system (shown here inside a ProMetric® I Imaging Colorimeter) enables a measurement device to capture light and color as perceived by the human eye.
Studies of recent display metrology systems and methods demonstrate the effectiveness of high-resolution tristimulus imaging systems—combined with sophisticated algorithms for calibration, registration, and measurement—to solve microLED display luminance and color uniformity challenges and support the viability of microLED technology for the display device marketplace. These studies, along with methods for ensuring consistent luminance and color performance of microLED displays, are discussed in an article recently published by MicroLED-info.com, Measuring and Correcting MicroLED Display Uniformity.
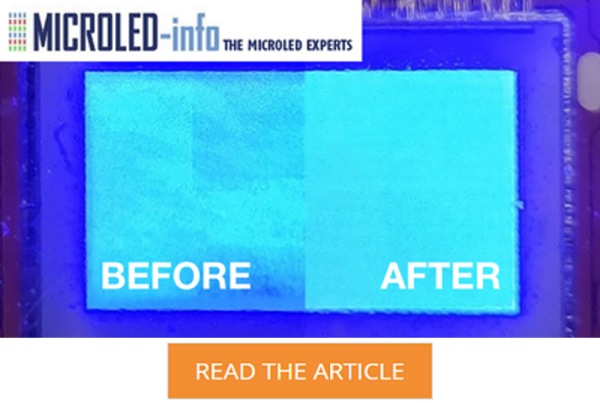
CITATIONS:
- Wu, Y., Ma, J., et al., “Full-Color Realization of Micro-LED Displays”, Nanomaterials, 10(12): 2482, December 2020. DOI: 10.3390/nano10122482
- “Micro-LED Introduction”, on MicroLED-info.com, last updated September 22, 2020. (Retrieved 3/18/2021)
- Chen, S.H., Huang, Y., et al., “Full color micro-LED display with high color stability using semipolar (20-21) InGaN LEDS and quantum-dot photoresist”, Photonics Research 8(5): 630-636, 2020. DOI: https://doi.org/10.1364/PRJ.388958
- Antolini, F., and Orazi, L., “Quantum Dot Synthesis Through Direct Laser Patterning: A Review”, Frontiers in Chemistry, April 17, 2019. DOI: https://doi.org/10.3389/fchem.2019.00252
- Kim, K., Lim, T., et al., “High precision color uniformity based on 4D transformation for micro-LED.” Proceedings of SPIE Vol. 11302, February 2020
- Carter, E., Schanda, J., et al., CIE 015:2018 Colorimetry, 4th Edition, 2018. DOI: https://doi.org/10.25039/tr.015.2018
Join Mailing List
Stay up to date on our latest products, blog content, and events.
Join our Mailing List